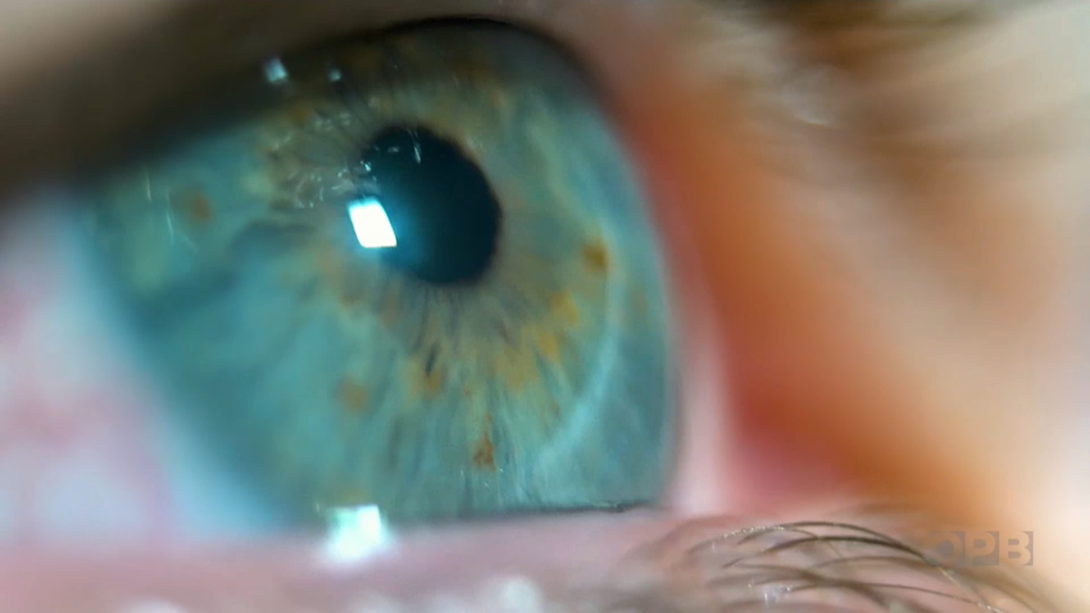
Coveralls: Check.
Boot covers: Check.
Hood, mask and gloves: Check, check and check.
Physicist Saba Moslehi is covered head to toe in protective gear and ready for a shift in the clean room.
“It’s not comfortable wearing that gear,” she said. " It’s hot, it gets hot.”
But the gear is necessary for Moslehi’s research.
“I can fabricate samples … without any dust or contamination getting into the surface,” she explained.
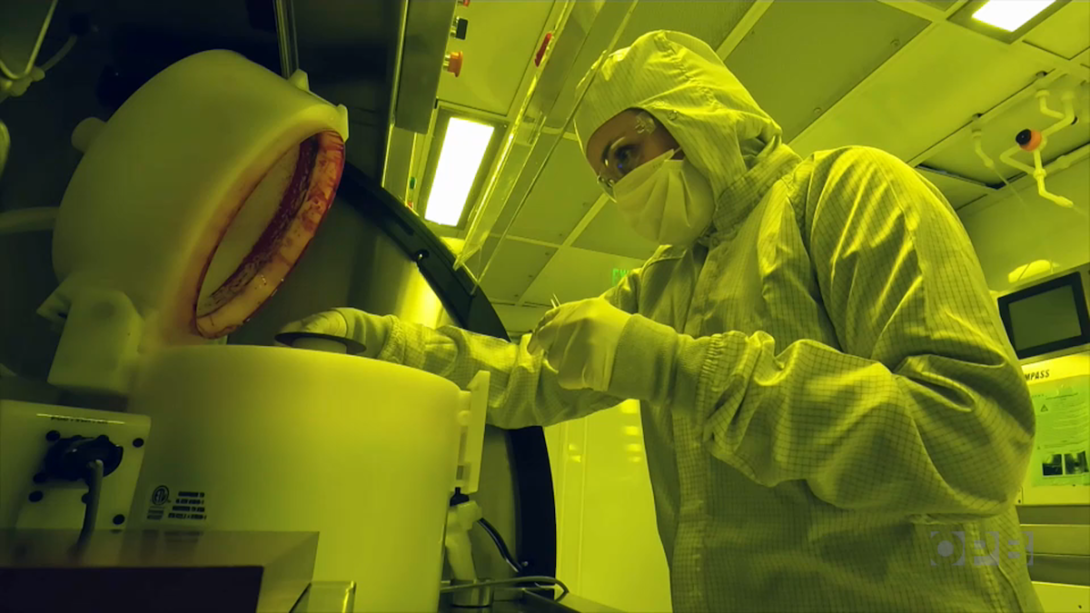
The “sample” she’s making in the yellow glow of the University of Oregon clean room is a small silicon electrode — thin as a piece of paper, but stiff. It’s designed to be a new interface for retinal implants, the part of the artificial device that brain cells need to connect to.
Their new design could eventually help restore eyesight to millions of people.
“Vision is our primary sense. It’s fantastic. It works beautifully — until it stops working beautifully,” said University of Oregon professor Richard Taylor, who heads the lab developing the new technology.
In the retina, there are photoreceptors that convert light into electricity, and then neurons carry that electrical message to the brain.
“There are a number of diseases that can attack the retina. And then you know what happens then is that gradually you start to lose your vision,” he said.
One out of every 10 people over the age of 50 will develop macular degeneration. Age-related macular degeneration causes your straight-ahead vision to blur because of a deterioration of the retina – the area at the back of the eye that’s sensitive to light.
Retinal implants are designed to reverse vision loss from diseases like macular degeneration and retinitis pigmentosa by replacing the damaged light sensors in the eye with artificial ones.
“The idea is that, long term, we will drop this implant in, which will have an array of artificial photoreceptors. Light will come in, generate electricity and then that will pass to the natural neurons that have not been affected by the diseases,” he said.
Retinal implants are already being tested in people, but so far, none have succeeded in significantly restoring vision.
“So we thought, ‘Ok, if the implants are not working, there must be a reason for that,’” Moslehi said.
The culprit, according to the researchers? The brain and the implant just aren’t fully communicating.
Bionic challenges
The idea of integrating electronics into the human body for medical treatments has been around for a while. The first pacemaker was implanted in the 1950s, the first cochlear implant happened a few years later, and more recently implants that provide deep brain stimulation have provided relief for people with epilepsy, Parkinson’s disease and obsessive-compulsive disorder.
But convincing biology to meld and interact with electronic components remains a challenge.
“What we are trying to do is to conduct electrical signals between the device and the biological components, right? The cells or the tissue. The keyword here is the between,” said Sihong Wang, a professor of molecular engineering at the University of Chicago, who is not connected to the University of Oregon research.
But Wang said the electrical signals can be disrupted and blocked by the body’s immune system.
“With electronics being typically very rigid, brittle, made with hard inorganic materials ... and also the chemical property has a very big compatibility issue with the human body. For example, once it’s implanted, it will trigger the immune system to react and then lead to the severe foreign body response and the inflammatory reactions,” Wang said.
For retinal implants, the device also must encourage as much connectivity as possible between implant interface and human brain cells, the neurons.
The Oregon researchers’ solution is to find a pattern and texture for the electrodes that the neurons want to attach to. And to find that pattern, Taylor is taking his inspiration from nature.
“A lot of the artificial electronics tends to have smooth surfaces. And smooth surfaces don’t exist in your body,” he said.
Smooth surfaces, right angles, clean lines. These are all the realm of Euclidean geometry.
“Straight lines, triangles, squares, circles, spheres, the things that we all learn are shapes when we’re growing up,” he said. “But out there, nature uses this very different geometry, a much more complicated geometry.”
Nature’s geometry is fractal. Think of the shape of a tree trunk and its branches.
“If you zoom in and look at the twigs, you’ll see that the same pattern is actually repeated, and it keeps on repeating and repeating at finer and finer scales,” he said. “Even when you look inside you, your blood system, your veins are fractal. The wiring, your neurons are fractal. … So really it’s the fingerprint of nature.”
Their idea is to meet nature with nature.
“What if we build an electrode that has a fractal shape, that sort of mimics the shape of the neurons?” asked Moslehi, recalling the question they posed at the start of the project. “Would that eventually end up increasing the resolution of the potential future implants?”
Like with digital photos, the better the resolution, the sharper the image.
“That’s our goal,” she said.
Encouraging connections
In the cleanroom, Moslehi starts by adding a special chemical called photoresist to the top of the silicon wafer. She then exposes the wafer to light with a laser writer.
“It changes its molecular structure, so that later I can wash those parts away and create a mold,” she said.
The mold will shape the layout of the electrodes on the chip, which will be made of highly conductive microscopic structures called carbon nanotubes. The design they’re currently prototyping is a fractal square pattern.
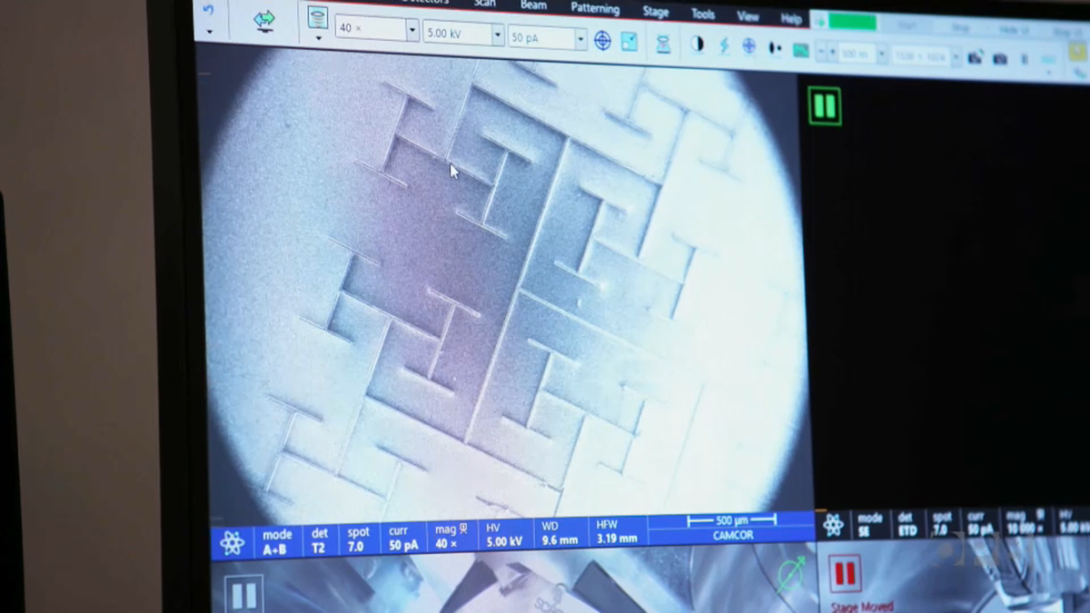
But it was an H-tree fractal that first demonstrated what could be possible through this line of thinking.
They were looking for a pattern that would encourage neurons to latch on, but also manage the neurons’ cellular groupies, which are called glia.
Glia serve as the bodyguards and life support of the neurons, but they have to be close by to do their jobs, and without them the neurons don’t function properly. When glia detect an unfamiliar object — like, say, a smooth implant in your retina — they tend to wall it off and prevent your neurons from making good connections. This is one of the immune responses of the human brain.
“Glia cells are like a non-conducting layer,” said the University of Chicago’s Wang. “Conduction can only happen through conductor. If you add an insulating layer in between, then it will make the conduction much worse. So that is a very common result of the immune reaction.”
This is where the H-tree is so successful. Moslehi placed the H-tree chip in a Petri dish with retinal cells from a mouse. Seventeen days later, she checked the progress. To her delight, she saw that the pattern and carbon nanotube texture of the Hs attracted the neurons, and the glia were funneled into the gaps in between.
“So the glial cells aren’t getting in the way of the interaction between the neurons and the implant. But the glial cells are close enough still to supply their life support,” Taylor said.
Their hope is that by combining that life support with better connectivity between the neurons and the artificial electrode, what the brain “sees” with the implant will be much clearer.
“The very first time that we imaged these samples under the microscope, and I saw a big cluster of glial cells within the gaps, it was like, ‘Oh my God, we did it. It happened,’” Moslehi said.
More fractals
Now that the University of Oregon scientists have a pattern that works, they’re starting to refine the texture of carbon nanotubes – the material the fractal pattern on the chip is made of.
The material is so small that a scanning electron microscope is needed to view it in any detail.
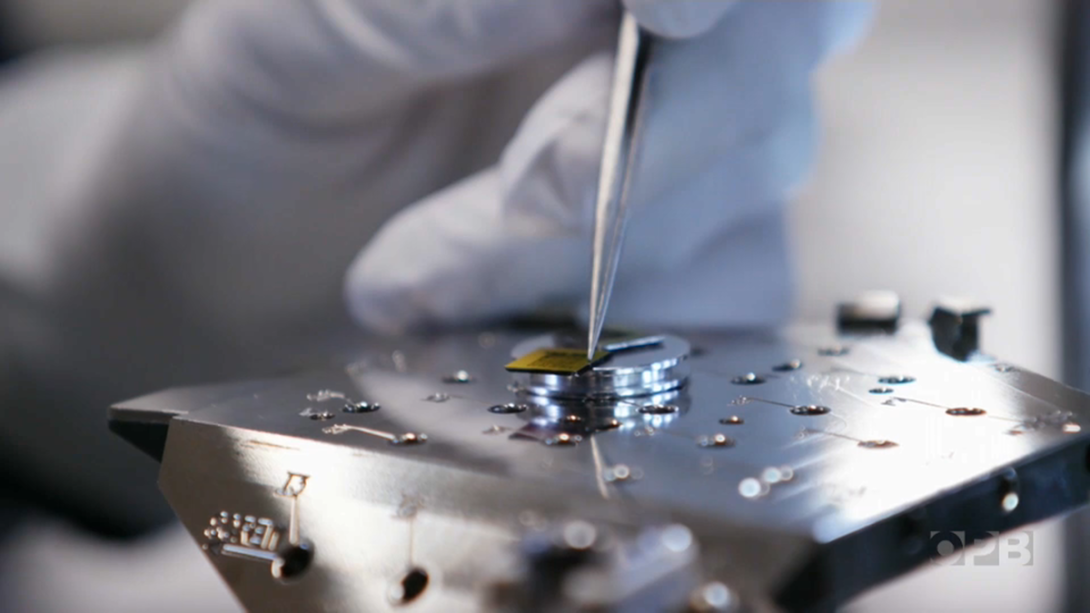
Moslehi places the chip into the microscope chamber and the H-tree pattern comes into view, filling the computer screen. Then she pushes in closer to see more detail.
“So right now I’m at … 20,000-times magnification,” Moslehi said. “This is the top surface of the nanotubes.”
It looks fuzzy — like the soft half of Velcro — millions of wiry hairs standing on end.
“The roughness of the top surface of the carbon nanotubes that we grew sort of gives the neurons this ability to adhere to the surface,” she explains.
She changes the camera angle, revealing the upper profile of the nanotubes.
“That mountain range-like structure,” she said, pointing out the tiny topography.
That shape is also a fractal - a more chaotic version called a statistical fractal.
Moslehi and Taylor plan to test different versions of this mountainous fractal pattern to see if they can encourage even more neuron connectivity.
“That is one of the wonders of science. That you can, just by … pushing those frontiers, you can actually do something very useful as well,” Taylor said.
“If we get this right, we could actually restore vision to somebody.”